New Motorized Tip Sp...
APAS® Can Screen an...
10th October 2016 Content supplied by: TSI GmbH
Real-time Viable Particle Monitoring: How Does it Work? How Can it Help?
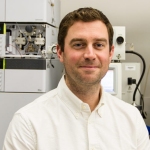
by Patrick M. Hutchins, PhD – Global Product Manager, TSI Inc.
For many decades the only practical metric of airborne microbial contamination has been the colony forming unit (cfu). Adapting growth-based approaches for monitoring modern aseptic manufacturing processes has sometimes proven difficult. Although microbiologists have bemoaned the limitations of measuring only cfu, no alternative metric or technique has yet been adopted. In this article we will review one such alternative microbiological method, commonly called laser induced fluorescence (LIF). For years LIF has played non-compliance-based roles in microbial detection such as root cause investigation and even military treat detection. In aseptic manufacturing LIF shows potential to replace growth-based methods in certain manufacturing paradigms, e.g. highly-automated, near-continuous processes. Here, we will review the fundamentals of LIF microbial detection, its benefits with respect to current methods, applications in aseptic manufacturing, and finally the regulatory outlook on LIF.
Viable Particle Discrimination: Laser Induced Fluorescence
Laser induced fluorescence (LIF) is sometimes referred to as enhanced active air particle counting, or auto-fluorescent active air particle counting. All of these names refer to the same core technology, which more closely resembles optical particle counting than traditional microbiology. In LIF, each airborne particle is interrogated independently and a determination is made whether an individual particle has characteristics consistent with a microorganism or benign airborne particulate.
To discuss LIF it is first necessary to have a basic understanding of optical particle counting. This is the ubiquitous cleanroom monitoring technology used to determine the concentration and size distribution of total airborne particles (generally >500 nm). Particles are sampled and directed through an optics chamber where they pass through a laser beam; typically a red laser. Each particle scatters the laser light as it passes through the beam. Light scattering events are enumerated and reported with respect to the volume air sampled, i.e. number of particles per volume of air. Moreover, the intensity of each light scattering event is recorded and this information correlates to the size of the particle, a phenomenon commonly referred to as Mie scattering. Thus, the optical particle counter reports the concentration and size distribution of airborne particles.
Like optical particle counting, LIF also employs laser light. To determine whether an airborne particle is viable or non-viable (i.e. a microorganism or not), LIF leverages the fact that microorganisms contain relatively high concentrations of fluorescent molecules. Therefore, when laser light impinges on a microorganism not only is light scattered but some of the light is absorbed and reemitted at higher wavelengths. This fluorescent light is distinct from Mie scattered light which occurs at essentially the same wavelength as the impingent laser light. As depicted in Figure 1, the intensity of fluorescent light is a critical characteristic for the discrimination of viable particles by LIF.
Figure 1. Simplified representation of the optical characteristics of viable and non-viable particles.
When a microorganism enters the LIF optics chamber it transits the beam of a blue laser (blue light better matches the average absorbance of the various fluorescent molecules in microorganisms). As depicted in Figure 1 on the right (VIABLE) two distinct optical phenomena occur. Some of the laser light is absorbed by fluorescent molecules and reemitted at higher wavelengths and some of the light is scattered. Both the scattered light and the fluorescent light are focused and filtered, before their intensities are detected. The intensity data is used to determine if the particle is viable or non-viable.
How this data is collected and how it is used to discriminate viability differs between instrument manufacturers. For example, in the BioTrak® Real-Time Viable Particle Counter model 9510-BD from TSI Inc., three different light intensities are measured for each particle; scattered light intensity, fluorescent light intensity from 430 to 500 nm, and fluorescent light intensity from 500-650 nm (excitation occurs at 405 nm). The three parameters are processed by a proprietary algorithm and a viability determination is made. The algorithm was derived empirically through the measurement of numerous microorganisms and inert particles. Figure 2 shows the value of measuring multiple optical parameters for discrimination. Panel A shows pollen and bacteria poorly discriminated by 2 parameters, panel B shows three optical parameters (as measured by BioTrak® model 9510-BD) providing discrimination between common viable (bacteria) and non-viable (pollen) particles.
Figure 2. Improved viable discrimination using three optical parameters. Panel A only shows two optical parameters, resulting in poor discrimination. Panel B shows markedly improved discrimination using three optical parameters. Both panels show superimposed data from Ralstonia Pickettii (red dots) and Bermuda Grass pollen (blue dots), collected on BioTrak® model 9510-BD (TSI Inc.).
Because optical analysis is non-destructive, total particle counting and viability detection can be combined with each other and/or other devices. For example the above referred BioTrak® model 9510-BD instrument combines total particle counting and viability detection. It contains two optics chambers, one for total particle counting and another for viability discrimination. The unit also captures particles on a gelatin filter device after optical analyses in order to support subsequent growth for identification and/or confirmation of positive results.
Potential benefits of LIF compared to growth-based methods
Microbiological techniques for environmental monitoring rely on capturing airborne microorganisms, providing conditions permissive for expansion and subsequent detection of the resulting colony. Whilst there are a myriad of permutations for this general scheme, including Rapid Microbiological Methods, their common reliance on growth presents a challenge for environmental monitoring applications in aseptic manufacturing processes. The next sections will discuss three specific aspects of microbial monitoring where LIF could provide significant improvements for aseptic processes compared to traditional growth-based techniques.
Real-time Data: The most obvious limitation of traditional microbial monitoring is the time required for culture. The longer the period between sample collection and detection of contamination the less actionable the information becomes. Certainly this issue has driven the emergence of numerous RMMs aiming to shrink the time period between microbial capture and detection. However, even rapid methods cannot achieve “real-time” results.
LIF-based methods offer “real-time” data. Data can be collected in the order of seconds or minutes depending on the configuration and operation of the instrument; sufficient for the results to be immediately actionable. The benefits of “real-time” process knowledge in critical environments are immeasurable. They include the ability to take immediate corrective action, to access and allocate risk, reduce product loss, and reduce sterility testing burden amongst many other benefits. “Real-time” data is also more easily integrated into automated facility monitoring systems, which improve data integrity and allows users to develop trends (see the newly revised ISO 14644-2).
Intervention Free: Increasing adoption of isolators in aseptic manufacturing is driven by proven performance. The fundamental advantage of these environments is their physical separation from human operators. However, these enclosed designs compel operator intervention for traditional microbial monitoring. This paradox puts risk reduction at odds with quality assurance: intervention increases risk but microbial monitoring requires intervention. Safe and effective microbial monitoring presents a growing challenge as human operators are increasingly excluded.
Since LIF does not require colony formation for microbial detection regular delivery of fresh media is not required. Moreover the unit physically resides outside of restricted Grade A areas such as isolators, in much the same configuration as optical particle counters. Line stoppages often lead to wasted product, sub-lotting, extra sterility testing, and exposed drug product, can all dry at the filling nozzle. Elimination of interventions for microbial monitoring avoids the associated risk and risk mitigation required following line-stoppages.
Continuous Monitoring: It is very difficult to achieve truly continuous microbial monitoring using traditional methods. Even if the environment is sampled continuously—in the case of settle plates for example—the data is not temporally resolved. Methods which do offer some degree of temporal resolution—slit-to-agar samplers for example—cannot operate uninterrupted for sufficient durations.
Again LIF provides a potential solution. The technique allows continuous sampling for hours, days, even weeks; certainly long enough to monitor a complete manufacturing shift. With continuous monitoring the data collected can be associated with specific events occurring during a process, material transfers for example. Continuous real-time data contributes to process understanding and allows operators to know the process is in control before, after and throughout.
Applications for LIF in Aseptic Manufacturing
LIF has the greatest potential to directly improve manufacturing processes when used for in-process environmental monitoring of the aseptic core. LIF instruments can replace active air samplers, settle plates, total particle counters or all three methods. LIF microbial detection instruments are used as a part of an automated facility monitoring system in these applications, which takes full advantage of real-time, continuous microbial data to impact and benefit manufacturing processes in several ways. Table 1 outlines the major impacts of replacing traditional method for environmental monitoring of the aseptic core.
Table 1. Impacts of LIF for in-process environmental monitoring
There are also several applications where LIF can easily be implemented and provide valuable data for users. The most obvious and widely implemented is root-cause investigations. Aseptic facilities spend a great amount of resources investigating microbial excursions. The time it takes to get microbiological results is a major challenge to completing closing investigations expeditiously. LIF-based instruments can scan areas for contamination rapidly by providing real-time feedback as the area is scanned. Also, continuous monitoring strategies can reveal excursions associated with transient events such as shift changes. LIF-instruments can even be used like a Geiger counter to quickly and precisely locate point sources of contamination. Similar functionality can be used to evaluate gowning practices, provide data for room release, and other activities where rapid data collection from multiple sampling locations is critical. Because LIF is often used in conjunction with compendial methods in these applications they can generally be implemented without undue regulatory scrutiny.
Regulatory Outlook
For LIF, or any alternative microbiological method, to impact real-world aseptic manufacturing there needs to be motivated adoption by industry and active support from regulatory agencies. It is widely known that regulatory agencies actively encourage implementation of rapid and alternative microbial methods, but what is the current landscape in industry?
It appears the benefits of LIF are resonating with aseptic manufacturers. Implementation for compliance based applications, such as replacing compendial methods for environmental monitoring of the aseptic core, is actively gaining traction. Specific case studies of in-process applications of LIF have not yet entered the public domain, although this may not be far off. LIF users have long begun the work necessary to validate the technique for in-process applications. Typically instrument vendors have played an active role in those studies including supporting validation efforts with Drug Master Files. One public indication that the pharmaceutical industry is actively working to adopt LIF is the recent article posted by the BioPhorum Operations Groupstating that LIF will be the first alternative method in their workflow, with the goal of creating an agreed-upon regulatory pathway for implementation (www.biophorum.com/article/167/establishing-qualification-pathways-for-rapid-micro).
Adoption of LIF for non-compliance based has applications, such as root-cause investigation, has been steadily growing since the technology has been introduced into the market. Several case studies have been presented and these application offer immediate benefits and promote familiarity with the technology. Companies and facilities which begin exploring LIF with non-compliance based applications have proven to be leaders in validating the technology for in-process applications.
Summary & Conclusions
Laser Induced Fluorescence (LIF), also called enhanced active air particle counting, or auto-fluorescent active air particle counting, is an alternative microbiological method for the detection of airborne microorganisms. LIF is not growth-based. Rather, it detects individual microorganisms in real-time, giving it the flexibility to improve aseptic manufacturing processes. By eliminating interventions for agar plate changes in the aseptic core and by providing real-time, continuous data, LIF has the potential to reduce risk while increasing manufacturing efficiency and capacity.
For further information on the BioTrak® Real-Time Viable Particle Counter visit:
www.tsi.com/biotrak-9510 or E-Mail: tsi.emea@tsi.com
Tags:
Date Published: 10th October 2016
Source article link: View
Related news
New Motorized Tip Spacing Pipettes
APAS® Can Screen and Sort